Organoids: how are in-vitro models revolutionising drug development and testing?
Invent: Life Sciences Episode 5
Speakers: Stuart Lowe, Sylvia Boj, & Bas Trietsch
[Music Playing]
Stuart: How can we trust that the medicines we take are going to be effective and safe? Well, we can take confidence from the rigorous clinical trials pharmaceutical companies undertake.
These trials, test the effects of every drug using human volunteers before release onto the market.
In order to better protect the people volunteering for these trials, regulators decreed that data from studies on animals would be the primary evidence needed before approving a clinical trial in humans.
This seemed like a reasonable, practical alternative until we began to see late-stage clinical trial failures on therapies that had passed the animal tests. Surely, scientists argued data from human-derived tissue samples would be much more representative.
Technology developers, being a creative lot, have been busy generating a range of in vitro models. For instance, organoids that develop into tissue like structures and organs on chips that allow us to engineer the cell environment.
Are these technologies in competition for a slice of the farmer development market? Or could data generated from different types of in vitro models all contribute to a bigger picture?
Join me, Stuart Lowe, as we plug into Invent: Life Sciences, a podcast brought to you by technology and product development company, TTP. Today, we ask how are in vitro models revolutionizing drug development and testing?
Representing a human organ in a model system is no mean feat. The model needs to be complex so that it simulates the functionality of an organ in vivo. But at the same time, we need simple-to-understand architectures in order to correlate drug effects with system outputs.
Creating an effective in vitro model is deeply rooted in thoroughly understanding the biology of the organs that you're trying to represent and how to manipulate cells to generate the correct phenotypes.
This is where many of the breakthroughs in the field have come from. And to find out more, I spoke with Sylvia Boj. Sylvia is the Chief Scientific Officer at Hub Organoids, and has spent years investigating and promoting organoid technology.
I asked her about what motivated her to join this field.
Sylvia: My name is Sylvia Boj, and I'm now the Hub Organoid CSO. But actually, I started my first contact with organoids as a postdoc in the lab of Hans Clevers. I was part of the team that developed the pancreatic models. Once [inaudible 00:02:56] had established the first organoid model.
And now in the company, we are focusing our efforts on translating this technology to be adopted by industry as a relevant preclinical platform for drug development and drug discovery.
Stuart: Excellent. Well, it’s great to have you on board and there's actually a lot to unpack. Could you tell us in kind of a simple possible terms, what exactly is an organoid?
Sylvia: The most simple way to define an organoid is that it's a mini organ, a culture in vitro. To provide a bit more detail, organoids are these 3D structures self-organise that recapitalize the physiology of the tissue that they're derived from.
And of course, they can be generated from embryonic stem cells, induced pluripotent stem cells or adult stem cells. And in our case, our companies focus on the technology developed by generation of organoids using adult stem cells.
Stuart: So, these are models of an organ but that can exist outside of the body?
Sylvia: Yes, so in these organoids, we have different cell types, and these cells are maintaining their function related to the tissue they're derived from, and they maintain the cell-cell interaction, so they're able to reproduce the physiology of the tissue they are derived from.
Stuart: Why has it been necessary to develop organoids as a technology? Is there some deficiency in the way that we've been doing things before testing on organs, which is sort of within an animal? Why do we need to isolate these from the rest of the system?
Sylvia: Well, I mean, an important characteristic of the organoids is that from one side, they're able to maintain the stem cells and the stemness of the tissue. So, this is what allows the organoid to be expanded in culture over time.
But at the same time, they also represent the differentiated cells, the older cells present on the tissue. When we just isolate primary cells, we are normally working with the differentiated cells.
I mean, if we think on hepatocytes for example, then we know that when they are in culture, they can be expanded or they can be kept alive for several days, but it does not have the right conditions to expand these cells.
So, with organoids, we are able to represent these cells in a way that they're expandable and we can work with them in the lab.
Stuart: Why is it that you might want to use an organoid in research? What is special about that sort of 3D in vitro model?
Sylvia: I mean, of course, when you work on in vitro models, you remove a lot of the complexity. When you try to study maybe the same tissue in vivo, it's also more easy to manipulate.
So, definitely in academia research, organoids have brought a new open of research in which people can understand better how cell-cell interaction works, how maybe stem cells, what are the pathways that induce the stem cells from one side to maintain the stemness, but also what pushes differentiation.
Also, now with the combination of great technologies like Cas9, you can perturb gene expression in this in vitro model and have a more depth understanding of the cells involved from the generation of this tissue.
And of course, one of the emissions of our organisation is then to translate this same platform in more application and to be, for example, a preclinical model adopted by industry for drug development. Because we also believe that organoids can bring a value that current preclinical models were not able to represent.
Stuart: So, key to the effectiveness of an organoid model is controlling how it's prepared in the laboratory. If this is done successfully, researchers can benefit from the reproducibility and convenience of these in vitro models.
In some cases, gene editing is used to make the organoids more representative of the disease state we wish to model, improving the quality of the insights derived from them.
In other cases, the organs we wish to model are best represented in a dynamic system, which is where organ on a chip comes in.
Organs on chips are engineered systems, typically microfluidic that allow organ models to be deployed and interrogated in a more physiological context, including external stimuli like blood flows and stretching.
Several companies are commercialising this modality. And I spoke with Bas Trietsch, Chief Technology Officer at Mimetas to find out more.
Bas: My name is Bas Trietsch. I'm the Chief Technology Officer at Mimetas in the Netherlands, also one of the co-founders of Mimetas.
And over the last decade, I've been trying to use my background in drug development research as well as microfluidics to push forward our capabilities in organ on a chip to help pharma find new medicines for currently intractable diseases faster and more efficiently.
Stuart: That must be a lot of fun. What exactly is an organ on a chip just for our listeners who don't know?
Bas: Organ on a chip is actually a way to build miniature versions of organs, of pieces of tissue. So, it can be miniature livers, miniature kidneys, miniature lungs, and we build them in a way that allows you to interrogate how these tissues respond to different stimuli better.
So, conventional research, you would take cells can be immortalised or cancer cells, check them in a petri dish, they grow there, they keep growing, and then you can use those to test drugs on. If I put a potential drug on it, how does it affect the cells in the petri dish?
And that's been incredibly useful. But it also has its limitations because those cells without a context, without a three-dimensional shape, without blood vessels, without perfusion, and also, without the different cell types working together to form an organ, they still have the same genetic makeup, but they really behave and respond differently to stimuli as a full tissue in the human body would.
So, organ on a chip is a way where we borrow technologies that were developed to make computer chips, to make printed circuit boards, where we've developed technologies to do microfabrication to precisely control where occurrence and electricity goes, and instead use that to very precisely control where cells and body fluids go.
And that's where the on a chip analogy comes from. We take cells, we put them in a chip, and instead of running the right currents through them, we manipulate the cells to be in the position where they are. We provide them with the right blood flow, with the right type of nutrients.
And by doing that, we allow tissues to form that are much more close to how tissues are in the body. And that means if you do experiments on them also the results that you get from there are much more representative of how certain therapies or certain potential drugs would affect the body and, in the end, would affect the patient.
Stuart: It's like a mechanical simulation of something that's going on in the human body. It sounds like organoids are a really important technology for helping you get from patient-derived cells into fully fledged organ on a chip models.
How much investment or how much interest do you take in the development of organoids as an enabling tool for your systems?
Bas: It's a very important aspect of what we're doing. The only thing we can do with the chip side of organ on chip is provide cells with the right environment and give them the chance to develop in the right way.
We have a large degree of control, but if we can have the best chip in the world, if we put bad biology in it, it will only ever get so good.
And organoid technology is one of the ways in which we can actually get the diversity of cell types, get cells that maintain a lot of the characteristics of their donor simply because they've never gone through this weird state of being attached to a bit of plastic or something like that.
You have a very clean and very representative way of taking biology from patients and putting them into a manageable system that you can actually do experiments and do research with, and that you can propagate, that you can make millions and millions of organoids out of a single donor or a single piece of donor tissue.
Stuart: It's a really interesting synergy between the two. You need that sort of preconditioning before the organ on a chip becomes fully effective.
Bas: Yeah, it's something that we focus a lot on. We've invested strongly in building up a network in working with hospitals, with academic hospitals, but also with commercial suppliers to get our hands on tissues from patients or healthy donor tissue because like I said, our model systems are only going to be as good as the biology we put in it. And the trick is to not lose the properties of these tissues anywhere along the way.
These cells are good when they're taken out of a patient. The trick is to not let them to deteriorate in something that in no way resembles the patient anymore. And organoids are a way to take stem cells to allow them to keep reproducing and replenishing exactly the same as they would be if they were still in the body.
It's so good that you can actually, for some tissues, you can take these organoids, stick them back into the body, and they will integrate into the live tissue again. This is something that works with gut organoids pretty well.
In mice, at least, it's been used as a treatment where if you have lesions in gut, you can just stick in gut organoids, and they grow in place.
Stuart: So, it's no different from the actual tissue itself then?
Bas: Indeed. And if it's good enough for the real gut, I think it's good enough for our model system. So, we really like those donor tissues to take them, stick them not in a live gut, but in the microenvironments provided by our organ on a chip platform.
And that means that you've maintained all of those properties of the donor, whether it's healthy or diseased, that give you the perfect points for testing your new therapies and your new treatments.
Stuart: That's as close as you can get really, isn't it?
Bas: It is really trying not to lose any of the patient properties along the way.
Stuart: From talking with Bas, I got a sense of the painstaking research behind the development of human organ on a chip models. It's clearly been worth the effort since now, we have the ability to produce many replica organs on chips from a single patient and increase our testing capacity.
Earlier in her career, Sylvia worked on developing the pancreatic organoid models for mouse and human tissue, making her one of the lucky few scientists that helped to pioneer this new technology.
Sylvia and her team's efforts have already produced amazing results such as using intestinal organoid models to help combat cystic fibrosis. Sylvia elaborates.
That intestinal model that you've created seems really powerful and allows you to explore these niches that you hadn't been able to do before. What else have you managed to do with that model? Has it found other applications?
Sylvia: Probably the most unexpected result of developing this model is that indeed, we already have been able to impact patient treatment, and this is in the area of cystic fibrosis.
Stuart: And what has that model allowed you to do with cystic fibrosis patients?
Sylvia: Cystic fibrosis is a monogenetic disease in which one gene is mutated, CFTR, this is an ion channel, and this ion channel is expressed in many tissues, among them, the intestine.
So, actually, with organoids we can evaluate if the CFT channel is functioning by what we call a swelling assay. This assay was developed by the lab of Jeffrey Beekman in the children's hospital here in Utrecht.
As soon as he learned about the developments of the intestinal organoid models in in Hans’ lab, he had this great idea that we could measure indeed that we could develop an assay in which by measuring swelling of the organoids, we could evaluate the activity of CFTR.
And this is the base of an assay that now, is giving access to patients with a rare or ultra-rare mutations in the CFTR gene.
Stuart: And how does the assay help those patients?
Sylvia: So, there is a characteristic of the cystic fibrosis disease, and it's that there are more than 2000 different mutations identified that impact the activity or the functioning of CFTR.
And actually, for some of the mutations, we can go from two patients in the world to maybe groups of 20, 30, 45 patients with the same mutation.
So, if we think about how currently patients get access to a drug, and these are the clinical trials, these trials required a large number of patients in which you do placebo and compound and do a lot of tests, and try to build numbers to show the effect of your compound.
So, for this group of patients, the current process of drug development and drug validation does not allow them to have access to compounds. But then with organoids, we can create patient avatars, we can test it in the lab, and we can see if this is a small group of patients.
So, this specific mutation responds to the available drugs. And actually, this has been the case.
In 2015, a Dutch guy (he was 15, 16 at that time), was the first person in the world getting access to treatment that was working for him thanks to an organoid test. Otherwise, he would have not had access to such a compound because indeed there are only two people in the world with this mutation, himself and his aunt.
Stuart: That's a really great success story for organoids. And I suppose the non-animal testing field must feel quite validated by that result.
Sylvia: Yeah, definitely. I mean, we think that animals are great and sometimes, there are certain experiments that need to perform with them because we need to have a full biological system with all tissues and everything, but at the same time, they don't represent the patients.
So, with the organoids, we can bring relevant patient material into the bench, and we are definitely working to see in what a space organoids can already replace or reduce the number of animals that are required to get certain drugs to move above the pipeline development.
Stuart: It's quite incredible what the team in Utrecht have been able to do for cystic fibrosis patients. Their treatment recommendations based on human cell models totally circumvented the need for animal tests.
In vitro models have proven to be effective representations of human in vivo response. Greater acceptance by the industry could revolutionise preclinical testing and hopefully, pull us away from the widespread use of animals in pharma R&D. I asked Bas, about the impact of organ on a chip on animal testing.
Do you want to just comment a little bit about how this impacts on the field of non-animal testing? Because obviously, you've got the cell-based models for early-stage discovery, and then this preclinical testing, which in the past or traditionally, involves a lot of animal testing.
Can you talk about some of the benefits of not using animals in this type of research?
Bas: Yeah, so I think there's a couple of angles to that. So, on the one hand, there is of course, the ethical part, the general imperative to reduce animal testing. That's just something I think all of us as scientists, we should want to abide by the three Rs.
We should want to reduce, replace, and refine to get the best data for your research without needlessly harming animals. But to be honest, that for me is only a small part of the motivation.
I think the biggest part is that doing drug development on animals means you are developing drugs for animals. If you are using a wrong species that has completely different biology, that has different chemistry, that has a different response to different drugs, you are not developing a treatment for humans.
And sometimes people say, “We've cured cancer and we've cured diabetes from mice already a hundred or a thousand times,” but those drugs simply do not apply to humans, otherwise we would not be talking about them now because they would no longer be incurable or at least a chronic disease.
And this is something that I believe strongly in. I think animal models will probably for a long time still have a place, and that is to really study the very complex interactions where you need a complete body.
Interaction between all different organs at that very high level where you want to see some really unforeseen interactions. Areas where you really need a complete animal or a complete human to see this system level interaction between different organs. I think we're aways away from that. I'm not a big believer of the human on a chip context or at least not in the next 5 or 10 years.
So, you're asking what does organ on a chip mean for animal models? I think organ on a chip is not just a way to reduce the use of animals. I think 9 times out of 10, it's actually a better solution.
Because it allows you to use human tissues and not just single human tissues, but a large number of human tissues. If you do it correctly, it's a very scalable solution. So, you can actually get large numbers of data that allow you to do proper science and effectively, you are able to do experiments that are just way more representative of a patient that you would have to test these drugs on down the line.
And the interesting part of that is actually that it's my firm belief that this does not only count for a replacement of animal models, it actually also counts for a replacement of simple cell models.
The conventional way of looking at drug development can be, you start off with very simple assays where you can test millions of compounds, and you just throw out 99%, then you take your million leftover compounds or your 10,000 leftover compounds, test them on simple cellular models. Take those a little bit more complex cellular models, then go to animals, then goes to humans.
So, the first time you actually get a fairly relevant human model is in the clinic. That's why the vast majority of drugs that even of drugs that actually at some point are tested in the clinic that are taken by a human still feel they don't make it to the market.
If you can take more relevant models, human tissues with the right context, with all the aspects that we can have in these models to the very early days of development to replace the simple assays and replace the animal models, it means that you are going towards the right drugs all along the way because this is not you honing in on a drug, it's choosing an avenue.
And if at any point down this funnel you take the wrong avenue, there's no way of returning back to the right avenue. You've gone off a tangent, if you do that based on the simple cell models or if you do it based on animal models, either way, you are using the wrong model, so you're developing the wrong drug.
And that's why I feel very strongly about trying to use the most representative human tissue and human disease models from all the way in the start to as late as possible in the pipeline to make sure it's the most promising and the most high chance of success drugs that actually make it into the clinic and hopefully, to patients.
Stuart: As Bas points out, we've become very good at treating the models of disease, we've generated in animals, which hasn't necessarily translated to more therapies for humans.
In vitro models introduce the link to human physiology earlier in the preclinical phase, and hence could help rule out what doesn't work sooner. But if scientists are going to use these models to improve drug testing, they'll need to cooperate with the regulators.
With the U.S. Senate recently passing the FDA Modernization Act, which authorised the use of certain alternatives to animal testing, there is hope the industry will now embrace the technology. I asked Sylvia for her thoughts.
So, you must have been quite pleased when you saw the FDA Modernization Act was signed?
Sylvia: Definitely. I mean, I think that this modernization is opening a new avenue and a new window for organoids to fulfil the gaps that already the industry and FDA is aware that animal models cannot address or solve or bring the right data to take decisions on certain compounds.
And I think the most obvious space is now that immune oncology is becoming a hot area for drug development. Animal models cannot represent the human antigens that are present in human tumours and towards a lot of companies want to target compounds or cellular strategies to treat this tumour.
So, this is definitely a space where FDA is at least saying that they are open to see data where they're not animals used to then evaluate efficacy or the response or the value of certain compounds to move into clinical trials.
Stuart: This would be using tumoroid models then?
Sylvia: Yeah, I mean we definitely see a space there also in terms of reducing animals. We can also develop organoids from normal tissue from any species. So, an approach also to reduce the number of animal especially depending on the mechanism of action or the interest to evaluate the toxicity.
Already the industry is struggling to identify what is the relevant animal model that can answer the questions that they have about toxicology because different animal models work slightly different.
With organoids, we can also guide this decision by identifying the right species that maybe can show the data necessary to move forward.
Stuart: But what is the best case then? Do you see the complete elimination of animals? Is that a mission statement for non-animal models or how do you see them coexisting in the future?
Sylvia: We have already examples of compounds, and it was published recently in Cancer Nature. We have the first drug developed by using preclinically only tumour organoids.
And this compound is a bispecific antibody that can recognize cancerous stem cells because it recognizes cells that express lgl5 and ETF receptor. And by blocking these cells, can reduce the growth of tumour organoids.
This is what was evaluated in the preclinical phase. And actually, on the recent AACR meeting that happened last week in Orlando, it was presented the first results of the phase one clinical trial for this compound.
And there are already very exciting results on showing how the tumour of patients has been reduced already on the phase one clinical trial when they're evaluating the toxicity.
So, again, depending on the mechanism of action of the compound, we can already force the development of new drugs not using animal-derived.
I think that in the area of oncology, PDX models, so this patient derivation of transplant models in which a mouse or is implanted a piece of human tumour and kind of expanded is also part of the standards that until now, industry was using again, also as a part of the package data to move compounds into clinical phases.
But yeah, we already have the first example where you can move a compound without … or at least with identification of the generation of this lead compound by using only organoids.
We are just exploring in what areas, again, in what type of cancer diseases, cancer types and mechanism of actions and organoid based platform will be sufficient to develop a relevant compound that successfully treat patients.
Stuart: It's an exciting prospect to see that the FDA has begun to lower the barriers for non-animal testing in preclinical research. It's the right thing to do ethically, but it also makes a lot of sense scientifically, as Sylvia pointed out.
And with greater freedom for investigators, we're starting to see in vitro testing models being used to inform clinical trials and improve outcomes for patients. I was curious to see what other innovations might be on the horizon. So, I asked Bas for his outlook.
How about things like 3D bioprinting? We've seen the Vizient bio got their drug approved for NASH off the back of 3D bioprinting data. Do you think that organ on a chip could be used to help with that therapy or is there something particular about that advance that meant that it was the right thing to do there?
Bas: So, I think organ on a chip and bioprinting are two very complimentary technologies. Of course, there's one thing that you can envision bioprinting for that doesn't really fit with organ on a chip, and that is really trying to build large tissues intended for implantation.
Sometimes if I talk about we can grow liver, people say, “Oh, that's great, then you can actually use it for people who have liver failure” — well, not so much because we actually made an effort to make it as small as possible.
We have thousands of them, which is great for science, but it will take a lot of time for any of those individual micro livers to make a dent to the beer you've drunk last night.
But in a way, 3D printing and organ on a chip stems from a very similar philosophy, and that is you need to have the right cells in the right spots, able to interact with each other in the correct way. And in organ on a chip, we use these channels to control where the different cells end up and how flows flow through them.
In bioprinting, you put them down one by one or at least you pattern them with your 3D printer to get the right cells in the right way, in the right context with each other.
So, I think the philosophies are very, very well-aligned and I think one of the ways that we've explored also is to actually take our system because it's very good at doing vasculature at profusion, grow a microvascular bed in one of our chips, and then actually 3D print a tissue on top of that.
And what then can happen is that you have that 3D printed piece of tissue being vascularized by that microvascular bed that you cultured in your chip, and in that way, you effectively hook up the 3D printed piece of tissue to a profusion system, but not one, but actually a hundred or a hundred per plate.
So, if you have a few dozen plates, you can have thousands of chips that are where you have this 3D printed tissue, fully profused, fully vascularized that enables you to take the best of both worlds.
And again, take another step on our eternal quest of making model systems that are as close as possible to the in vivo situation.
Stuart: I like your comment about the best of both worlds. I suppose what do you see as the prospects for the world of 3D cell models, if we were to have this recording in 5 or 10 years, where would you like to be?
Bas: Oh, 5 or 10 years is a long ways away in this field because stuff is moving fast. What I hope to see is that we will have a pretty well-filled and well-fleshed out ecosystem in which there are established disease models for all of the different organs for a lot of the different diseases that are being studied.
And that there is a well-accepted way of going about it for the level of complexity that you need, that there is no longer everybody going every which way for their own question, but that this technology has become commodity enough that like if you want to do an ELISA, you don't think about all of the rocket science that’s happening under the booth.
You just use it to answer a question. And I think the moment our technology becomes boring, that's when I think we've been hugely, hugely successful because that means that we are actually focusing on the aspects that matter and that is understanding new biology.
And I think 5 or 10 years from now, I would really think that the majority of drugs that are reaching the market or in the process of being filed will have been made possible in one way or another by 3D cell culture.
It can be early stage, can be late stage, can be organoids, or can be organ on a chip. But I firmly believe that this technology will be ubiquitous, and I think you should no longer be seen as the brave person embracing this new technology.
We're already going there, but actually, you should be asked to explain yourself if you've used inferior models rather than the ones that are available using 3D cell culture, or using organ on a chip technology.
So, I think fully embedded in as a standard go-to way of understanding diseases, that's where I see organ on a chip in 3D cell culture in 5 or 10 years.
[Music Playing]
Stuart: Human disease is incredibly complex and thanks to the amazing work of life scientists, the pharmaceutical industry has developed and commercialised therapies to treat a vast range of diseases.
And to develop the next wave of therapies, we'll need to go deeper with our understanding of disease-biology. And the legacy methods of drug development using animal models will soon start to look out of date.
In vitro models like the organoids and organs on chips we heard about today, have the potential to provide drug developers with richer, more patient relevant data. As we heard from Sylvia's cystic fibrosis example, the use of in vitro models is already starting to benefit patients.
And with the innovations led by organ on a chip developers like Bas, the range of diseases that we'll be able to model is only going to increase. Regulators are starting to take notice too and are lowering the barriers for adoption in preclinical research.
Organoids, organs on chips and other in vitro models are already proving that we do not need to depend exclusively on animal testing to develop effective drugs. Industry and regulators stand to benefit from the increased predictive power afforded by these models.
If Sylvia and Bas are right, then their models could move from cutting edge to commonplace within a matter of years, which should, ultimately, lead to better outcomes for patients.
That's all for today. Thanks so much for joining us and a huge thanks to both Sylvia and Bas for sharing their expertise with us on this episode.
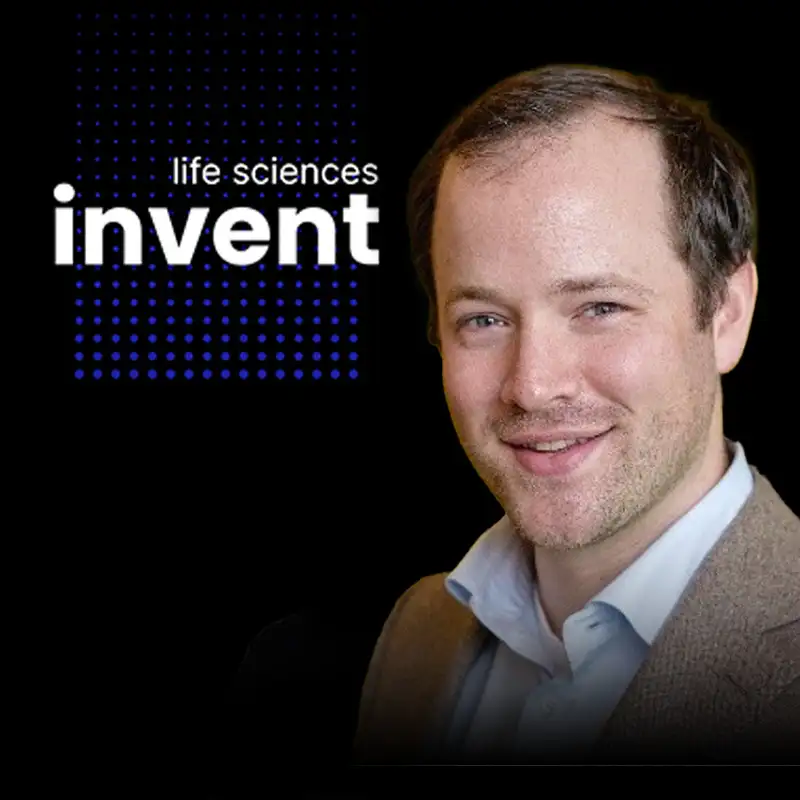