The Blood Brain Barrier: can innovations in delivery help us unlock the brain?
Invent: Life Sciences Episode 4
Speakers: Stuart Lowe, James Choi & Sam Barker
[Music Playing]
Stuart: Modern medicine has helped us develop many effective treatments for diseases found throughout the body, but we've yet to have the same success with the brain. Even as great strides are being made with treatments for cancers, the incidence of neurological disorders continues to grow.
Progress is being made, however, with promising results from phase three trials for Alzheimer's treatments announced early in 2023. But fundamentally, the brain is a difficult organ to access, both for conducting scientific research and for delivering therapies.
One of the challenges we need to overcome is the so-called blood-brain barrier formed by the tight junctions between the brain’s endothelial cells. This barrier controls the passage of molecules out of the blood and into the brain, and so it's not as easy to use the bloodstream to carry therapeutic molecules as we might do for other diseases.
Could the blood-brain barrier be the key to treating neurological disorders? How can we target this structure to make therapies more effective? Join me, Stuart Lowe, as we plug into Invent: Life Sciences, a podcast brought to you by technology and product development company, TTP.
Today, we ask, can innovations in delivery help us overcome the blood-brain barrier? Delivering drugs to the brain has historically been an invasive and risky process. It wasn't too long ago that the best modern medicine could offer was drilling a hole in the skull and administering the drugs that way.
Are we still dependent on these extreme techniques, or have we developed gentler methods? To find out, I spoke with Dr James Choi from Imperial College London. James leads the Noninvasive Surgery & Biopsy Laboratory and is an expert in the blood-brain barrier.
James: I'm James Choi. I run a research laboratory developing non-invasive surgical technologies, and one of the main focus for me is to develop a device that can deliver drugs to the brain.
I've been working on this kind of technology and different variations of it since 2004, and I started my laboratory here at Imperial in 2013. So, it's around 10 years from which I've been at Imperial.
Stuart: How big's your research group at the moment?
James: Right now, it's around six PhD students.
Stuart: And all working on different aspects of your technology?
James: Yes, exactly.
Stuart: Nice. So, one of the things that our listeners probably want to know is what is the blood-brain barrier and why are we trying to overcome it?
James: So, the blood-brain barrier is a very specialised interface along your brain's capillaries, so your tiniest vessels, and it regulates what goes in and out of your brain tissue.
And normally, this is a very good thing because it keeps all the bad pathogens and unwanted compounds from entering your brain space, but becomes hugely problematic when you have a disease such as Alzheimer's disease or brain cancer developing in the brain tissue because the drugs that are administered into the bloodstream cannot exit the blood-brain barrier and enter the brain space.
And so, we have a whole history of failed clinical trials for many reasons, not because the drugs aren't effective, but because those drugs cannot cross a blood-brain barrier and enter the brain parenchyma, which is where the disease resides at sufficient concentrations.
And so, it's a huge medical challenge, it’s one of the greatest medical challenges of our time. And a lot of people are really working hard to resolve this.
Stuart: So, what is the kind of the state of the art? What's the gold standard for gaining access to the brain at the moment?
James: There is no great method for delivering drugs to the brain. And so, this is why it's such an incredible challenge. I think people sometimes attempt doing direct injections, so they will drill a hole into the skull and directly implant a catheter so they can directly administer the drug.
But obviously, that has very huge problems where you're causing collateral damage enroute to the disease side that you want to treat. And then after you actually do the injections, it doesn't quite go where you want it to go because it tends to go up the shaft in the hole that you actually administer it.
But to be honest, the most common approach is just to inject a higher dose of the drug that you want into the patient. And obviously, one of the big problems with that is that you start having collateral damage or side effects in your eye, in your liver, your kidney.
And so, it becomes a huge problem because you cannot get a high enough dose into the brain tissue because then you have liver failure or different side effects that will end the clinical trial.
Stuart: So, it's actually more about the small amounts of drug reaching across the blood-brain barrier, and actually you can't get it to where it's needed. And I suppose that's an advantage when you're trying to prevent large concentrations or large fluctuations in concentration going across into the brain?
James: Exactly. I mean, the brain needs a very strict metabolic and ionic soup to function properly. You think about consciousness and memory and all these things require very strict environment so that your neurons can fire in a very intricate pattern that can kind of provide these types of features for the brain.
So, the blood-brain barrier is a good thing, and so we don't want to get rid of it. A lot of methods are just trying to bypass and circumvent it or temporarily change the blood-brain barrier permeability so that you can then have this short window in which a drug can actually enter the brain tissue.
Stuart: Yeah, I suppose it's kind of not … you don't want to subject it to some of the variability that you see just in the bloodstream right after you took in a meal, for example. You had a chocolate bar, suddenly your brain starts going haywire.
James: Surprisingly, well glucose thankfully gets into the brain because you need the glucose, the sugar to provide the energy for your cells in your brain tissue, so that that does go across.
Stuart: As James explained, the blood-brain barrier controls the passage of biomolecules into the brain and moderates the careful chemical balance within. Surgical techniques to circumvent the barrier are typically what's used for delivery.
But with the risk of failure and the highly invasive procedures, you're not going to get too many chances to deliver your drug.
Importantly, James mentioned that the barrier does allow for vital chemicals such as glucose to enter the brain, and it made me curious about this pathway and how it could be exploited to overcome the blood-brain barrier.
So, to find out more, I spoke with Sam Barker. Sam is the Chief Business Officer at SomaServe, a genetic medicines company that is developing new formulations to facilitate passage across the blood-brain barrier.
Sam: So, my name's Sam Barker, I'm Chief Business Officer at SomaServe. We are a Cambridge based biotechnology company, and the focus of the company is to enable delivery of new therapeutics using our technology, which is called PolyNaut.
Stuart: Hopefully, our listeners know a little bit about drug delivery, but maybe not about the intricacies of the blood-brain barrier. So, why is it a barrier? What are we describing here?
Sam: It's quite a fundamental protection to our brain, essentially. So, I think most of us know that our brain and the cells within our brain are really energy hungry. So, for a small amount of tissue, they use up a huge amount of our total energy consumption.
And so, that means that the brain is very highly vascularized with a lot of blood capillaries in there. But the brain tissue itself is also very sensitive. And so, the blood-brain barrier exists to regulate essentially that environment.
So, that can be nutrients being taken up from the blood into those cells like neurons, but it can also be the exit of waste, and then regulating things like fluid and ions. So, it is really important to protect the brain tissue from all of those things that are circulating in our blood.
And usually, those molecules circulating in our blood are able to diffuse fairly freely into our tissues and organs, but if they were to do that to the brain, they would severely disrupt our sort of cognitive function and brain health.
Stuart: So, it's like a buffer, almost kind of like a delay mechanism that means we don't get too many spikes in concentration.
Sam: Exactly. It's a highly selective membrane. If we think about the capillaries, then typically our capillaries aligned with epithelial cells, and they have gaps between them that allow molecules to diffuse and pass through. And they also have active mechanisms for moving biological molecules through.
If we look at the blood-brain barrier, those endothelial cells have got what we call tight junctions. So, they're welded together essentially, and they have no space between them for those molecules to pass.
Stuart: So, a completely different design almost.
Sam: Yeah, exactly. It has its roots. You can see the roots in the cells in their sort of phenotype from early on, but you can see that they've adapted over time to kind of create that protective layer that's highly selective.
Stuart: And what do you know about the history of neurological disorders? So, how people have tried to treat diseases of the brain in the past?
Sam: Well, I think it's entering a new era to be sure of that. So, previously I've worked with companies in the sort of neuro-oncology space, like brain cancer, so thinking about glioblastoma, and then in paediatric cases, you have a disease called DIPG.
And these are really difficult diseases, really difficult cancers to treat, especially for DIPG, which predominantly affects children around sort of age nine, have very low cases of survival, if not zero, you end up with incredibly invasive treatments.
So, there's obviously neurosurgery to try and remove the tumour, but when they're very infiltrative cancers, so it is very difficult to separate the healthy from cancerous tissue.
And so, there are various different technologies that have been developed for things like packing, different chemotherapeutics into those wounds or for direct delivery using pumps and intrathecal injections.
But one of the challenges with the brain is that it's actually very difficult to deliver to a single spot and to have a good diffusion throughout the brain.
Stuart: Is that related to the vasculature being quite tight then?
Sam: Yeah, it can be, but it can also be due to the sort of positive pressure forces. So, if you have a tumour growing in a brain, your brain is constricted by your skull, there's actually not much room. So, that can also make it difficult.
So, some companies have been developing technologies like convection enhanced delivery, so that's actually delivering the drug under a pressure.
And it's only in the past sort of couple of years where we are trying to expand delivery beyond just having to do direct brain delivery to think about how we can develop drugs that are more able to be delivered systemically, so through a vein, but then are able to pass across the blood-brain barrier and into those tissues in a therapeutically effective concentration.
And there's always been some small molecules, but as the field moves away from small molecules and towards more targeted drugs, that's where the challenge is laid.
Stuart: You don't really want to be doing brain surgery every time you want to deliver drugs to the brain, right?
Sam: Yeah, exactly. It's acceptable if the choice is that or a really severe disease. But we are trying to tackle diseases that are less acute nowadays and try and find more patient acceptable treatments and things that are also less of an economic cost.
Stuart: So, you don't need a surgeon present. You could deliver it just like a regular injection, perhaps.
Sam: Exactly. It could be a hospital infusion.
Stuart: And is that the route that you are targeting at SomaServe? Is that the mode of delivery then?
Sam: Exactly. So, the technology that SomaServe is developing is called PolyNaut. It’s a polymer nanoparticle, so it's a nanosphere made of co block polymers around a hundred nanometres in diameter.
But we're able to functionalize the surface of the nanoparticle with ligands that engage with cell surface receptors on the blood-brain barrier, and they trigger a mechanism called transcytosis, which is where those blood-brain barrier endothelial cells create a passageway to transport the nanoparticle from one side to the other.
So, we are using that to encapsulate new drugs, typically gene therapies, and to deliver them into the brain.
Stuart: Sam's PolyNaut technology utilises the transcytosis mechanism that allows transport across the blood-brain barrier. I wanted to know more about the genesis of the technology and how SomaServe are seeking to apply it.
And given the price of some gene therapies, I wondered how this delivery method might impact on the cost and what it might take to reach the market. Here's Sam again.
Why did you land upon polymer nanoparticles as a delivery mechanism? Were you looking for some other phenomenon and you landed upon the brain? Or was it always been the brain is where I want to go and this is the way to do it? What came first?
Sam: With that invention really predates my time with the company and as many biotech companies, SomaServe started as an academic group, and we've spun out from that. So, our academic founder, Professor Beppe Battaglia was at University College London, and he describes himself as kind of an interface between chemistry, physics, and biology.
And so, I think he's often led by invention and discovery. I mean, he’s certainly recognized the importance of brain delivery, also the kind of the different ligands that could be used, but also building on some of the information that was already in that sort of academic space.
And then it's been a process over the past couple of years as we've spun out from that academic group and into a biotech company to decide on what's the most commercially valuable but also what's going to give us a quicker route to the clinic so we can exemplify the technology in patients.
And so, it has to be that balance between addressing an unmet clinical need and then exploiting the invention, which I think is kind of where it came to. And then there is a real wealth of proof-of-concept data in other tissues, like in immune cells or cancer cells, which I'm also hopeful that we'll be able to exploit, but we might just have to do that slightly later down the line.
Stuart: And I suppose the beauty of gene therapy is that you can leverage platform technologies where the mode of action is known. You know that if you can get these into the cells, then it will have an effect and you know which genes to target, even if it's not the same ones that you would be targeting for blood cancer, leukaemia, or anything like that.
Sam: Yeah, exactly. There's a concept that we can design a vehicle that will deliver to a specific cell, and then the actual sequence of the nucleic acid within is interchangeable. So, you can address multiple different diseases.
And I mean, coming back to those smaller indications, those rare diseases, again, they're often limited by the cost of developing a new drug. And whether that is whether the population of the prevalence of the disease can actually weigh that up.
So, if we can have a sort of redeployable targeting bicycle that we can then use different cargoes for, there's that possibility that we can tackle multiple different indications without having to reinvent completely.
Stuart: But there's been other attempts at doing delivery of genetic or nucleic acid cargo. How does this stack up?
Sam: The most comparable would probably be lipid nanoparticles. Lipid nanoparticles are very much limited by liver uptake and ability to target elsewhere. They're also more sort of fragile or more prone to breaking up in solution and deploying the payload in the wrong area.
The most commercially advanced or towards the clinic, the most advanced is use of viral vectors. So, AAV vectors, and over the past couple of years we've seen AAV9, which is a specific vector which is able to deliver to the brain.
That has been quite transformative, I think, for the industry because it's the first true gene therapy that has made it to patients, certainly for neurological CNS diseases. And I think that will open the floodgates, really.
It will exemplify that it can be developed and administered to patients that it's safe and it's effective, but it will also highlight the need for more advanced delivery technologies.
So, these AAVs are kind of limited by firstly their costs and the difficulty to manufacture, but also, they're limited by the size of the payload that they can deliver.
So, if we want to deliver an entire construct, like a huge protein to do enzyme replacement, they won't necessarily have the space within that vector.
Stuart: So, an AAV is kind of a viral capsid with a defined volume inside, right?
Sam: Yeah, exactly. So, for smaller constructs they're really good, but as we get towards larger proteins, there's going to be limitations to how much you can pack in.
And then the other main issue with viral vectors is that they are seen by the immune system. Effectively, they're one dose and anything further than that, you'll have antibodies against them, and they'll be ineffective.
So, what we are trying to develop is a drug that can be multiple dosed. And so, for chronic diseases, we can give dosing over the lifetime.
Stuart: So, we've heard a lot about kind of gene therapies being cures, and so that is like a onetime only shot, right?
Sam: Yeah. And for some indications that works if you can recover that protein that's missing at a crucial stage in development. So, I think the reference there would be Zolgensma, but I think that's delivered to infants under two, and we don't yet know what the lasting effect is going to be there of that gene replacement.
And so, if we want to broaden it to more diseases, to more indications, then being able to perhaps do transient delivery for chronic conditions is going to be more acceptable and perhaps easier from a safety point.
Stuart: This might be a bit of a mean question, but what sort of price point would you expect a PolyNaut enabled gene therapy to land at? Or maybe need to target? Are we talking about millions as well, or is it a slightly different equation for you guys?
Sam: I think that we are targeting similar to lipid nanoparticles, the polymer process is a synthetic chemistry polymer manufacturing process with a relatively small footprint.
And then I think what we're going to find over the next couple of years is that nucleic acid drugs are going to become more and more commoditized, as it were, they're going to come down in price.
But from where we stand now, as a series A biotechnology company, that's something that you really can't calculate with any degree of confidence until you have really started manufacturing scale up and started talking to the regulators.
Stuart: I think your comment on the trends is probably indicative of where it's going though. I mean, if you are thinking about multi-dose chronic disease, then obviously the price has to come down if the payers are going to be willing to pay for it as well.
Sam: Which is ultimately what it comes down to. But typically, we are focusing on choosing indications that are going to make the most difference. And so, that will include some rare diseases where there might be higher price points.
But also, I would hope that targeting those big CNS problems like Alzheimer's, dementia, Parkinson's will also be within scope.
Stuart: Is that something you are targeting right now or is that kind of in the next wave or the third wave?
Sam: We operate a sort of a dual business model. So, we both develop our own internal programs, but we also work in partnership with Pharma. One of the benefits for that is that some diseases are relatively easy to develop for and that does include those rare diseases where you have a relatively defined patient population.
But what we found the industry has seen is that Huntington's, Alzheimer's, they are really complex diseases. The clinical trials are incredibly expensive, and you get a lot of late-stage failures.
So, in those cases, it does make a lot of sense to partner with Pharma who've got the experience and the resources. So, typically, we provide the targeting and the nanoparticle, they provide the cargo and a lot of the insight into the disease biology.
Stuart: So, partnerships are really important to you then?
Sam: Yeah, they are. We've always taken the approach and I have with previous companies as well, that as an early-stage company partnering certainly with larger organisations and with academia and biotech is really important.
Specifically, partnerships with Pharma are very good for validating the technology. They lend some weight to what you're doing, and that can help with investment. But also, they bring incredible resource behind them. So, you have access to their scientists, to their different manufacturing insights.
And so, it's a very symbiotic relationship. We bring something new to the table and they bring lots of experience and credibility with them as well. Just means that we don't have the same degree of control over it in terms of how that program progresses. But it's a really sort of helpful point for us.
Stuart: I could see why Sam was so excited about the potential of the PolyNaut technology. Having seen the impact that gene therapies like Zolgensma have had on neuromuscular disorders, imagine the impact they'd have if we could treat the brain directly.
Sam also highlighted the importance of partnerships in this field and the way that pharmaceutical companies have come to depend on innovations from outside to achieve their goal of delivery to the brain.
Given the delivery challenges we've spoken about, it's not surprising that several different methods are being investigated. Another promising method relies not on chemistry, but instead on a physical phenomenon to provide access. And I spoke to James again to find out more.
It's probably a good time to ask you a little bit about your research and what you're actually doing to overcome the blood-brain barrier in your group.
James: So, the way we've approached this problem is very different than probably your traditional drug modification technologies where you put the drug in a cargo. We use microbubbles and sound. It sounds very strange, but I'll walk you through it.
So, what we're trying to do with the microbubbles and sound is to modify the permeability of the blood-brain barrier. And the way we do this is we inject the microbubbles into the bloodstream. They're about the size of red blood cells. They're already used for ultrasound contrast imaging.
So, they're clinically approved agents, a few macrons in diameter, and they'll freely flow in and out of the blood vessels. These on their own do nothing. So, they do nothing. They will freely flow into your brain capillaries and out. We then apply a focus pulse of ultrasound onto the region where the disease resides.
And so, the bubbles within that acoustic field will then experience an oscillation. So, it'll expand and contract due to the acoustic pressure field, mechanically opening the blood-brain barrier, and then allowing the drugs within the bloodstream to come out.
And so, the really cool thing about this technology is that the focused ultrasound that is being applied is completely noninvasive and localised. So, you don't have to cut open the skin, the skull's completely intact, we can just focus the sound through the skull onto the region you want to target. And only in those regions do the microbubbles open the opening barrier and release the drugs into the brain parenchyma.
Stuart: That sounds really powerful actually. How large do the bubbles get when you excite them?
James: So, we're only discovering that right now in my laboratory. So, we have these microbubbles and we put them in these animal models of the blood-brain barrier. And so, we know that they can oscillate up to maybe twice or maybe three times its size within that confinement. But we don't have the exact figure for what is actually happening in human tissue.
Stuart: So, that's part of your research, part of your ongoing investigations?
James: Yes. And the oscillations can be quite mild too, so they can be maybe like 10% more or 25% more. It really depends on how much you're driving these bubbles with the ultrasound.
Stuart: And you've got quite fine control of it then.
James: Yes and no. So, that's one of the current challenges in clinical studies is to make sure that you don't oscillate them too much and you just do it at the right amount.
And so, we hit them with these pulses, and then what we do is we actually listen to how they sound, and based off of the unique ringing or sound that they emit back to the transducer, we can then adjust the next pulse.
So, it's like a remote-control system. So, we're emitting and listening, emitting listening, and we're trying to get these bubbles to oscillate at just the right amplitude.
Stuart: And can this be done just in the doctor's surgery, or do you have to go and be hooked up to a drip or something like that?
James: So, the micro bubbles are administered currently by drips. So, you take an IV bag and you put the micro bubbles in there and you drip it in. But people are trying to find better ways of administering that.
The drugs can be done by drip as well, or direct bolus injections. And each device that's being developed right now has their own approach to actually doing this.
Stuart: And then the transducer and the detection equipment sort of sits alongside the patient then?
James: Yes. There's different models, the different ways to do it, but one of them looks like a hair salon heater, so if you want to get like a perm, it kind of looks like that. And then other ones just look like a large disc that's near your head.
Stuart: James's innovative approach is a good example of the power of non-invasive techniques. Instead of going into theatre to have an operation on the skull, his infused microbubbles can form a precise opening in the blood-brain barrier. And all this can be done in a doctor's office.
I wondered whether James had thought about partnerships between startup companies and pharmaceutical companies to leverage his delivery technology.
James: At the end of the day, we build all these technologies, and we invent them in academic institutions and the government, and I guess society at large wants to find ways to take these technologies and bring them to patients.
Now, academic institutions do not have the monetary power and the I guess, focused attention to run such large clinical trials.
Stuart: They're not set up or organised in order to be a commercial.
James: So, to get this out to the patient, what you have to do is you either spin out a company or you've licensed the technology to a company and work with them to get that technology into the clinic.
And so, that's a combination of many things and relationship building. And at some point, the pharmaceutical companies will have to come into play and work with these companies to translate their technologies.
Now, some pharmaceutical companies may be interested in working with the academics directly just to get a quick feel or test to see whether their drugs are actually working in animal disease model, for example.
And so, there's no one road to translation. It's this kind of web of interactions between many different people trying to push it forward. And so, it's just a matter of will on the side of the device company, the pharmaceutical company, and the academics who are going to work together to translate this.
Stuart: And what's the most promising avenue at the moment that you're exploring or that's active at the moment?
James: So, these clinical trials by companies like Insightec, Cathera, NaviFUS, a bunch of other startup companies that are coming online soon, or they have products that they're working on that they haven't tested on human patients just yet.
And I would say that the three that are testing things on human patients, they're very promising. I'm very encouraged by those developments. It's not to say that there aren't problems, there are problems that that will be addressed.
Me specifically, as an academic, I'm trying to work on the second wave of technologies that will come in to kind of increase the usage of this technology.
And so, my laboratory focuses on developing safer, more robust, more reliable technologies. And this includes modifying the microbubbles, the pulse sequencing, the devices themself.
And so, the goal is to treat not just, I guess, life-threatening diseases such as brain cancers, but also target Alzheimer's disease, which you can imagine that the patient will need to come in once, twice a week for many months.
And so, what you want is a procedure to be very fast, very reliable, and extremely safe. So, you don't have any concerns of debilitating side effects that come with it.
Stuart: The risk benefit equation has changed at this point, right?
James: Yes, exactly. And then you start thinking about headaches, migraines, pain, you could use it as a local anaesthetic. You could use this technology for rare diseases like Rett syndrome. That's something I'm highly interested in.
And so, there's an immense amount of applications that we could explore. And scientifically, there's a lot of interesting questions as well to use this tool to figure things out. Like where is consciousness in the brain? Where is it localised? And would use this type of device to figure that out.
Stuart: James believes that his technology has a great potential for patients, and he is even thinking about what the next generation could look like. As he points out, if we can control access to the blood-brain barrier, we might even be able to discover new treatment pathways that we hadn't conceived of before.
So, what does the future look like once we've successfully navigated the blood-brain barrier? I asked Sam for his thoughts.
Imagine that the blood-brain barrier is not really a barrier anymore because we've got good ways of delivering to the brain. What opportunities do you think that opens up in medical sphere?
Sam: I think that's still going to be quite a major challenge. I think that with all drug development and all of the recent drugs we've seen come onto the market in say, the past 5, 10 years, they're not making such great leaps these days. I think that the blood-brain barrier will always be something that needs a specific tool.
And though I would love PolyNaut to be a comprehensive fix, we know that there will always be limitations. Now, having said that, like I said earlier, there are a wealth of different diseases to work on that affect people.
And also, if the delivery technology is able to be deployed really easily, and we can just change that genetic cargo, it means any disease with a genetic basis should be tractable.
I mean, people made those promises though when the genome project came out and it hasn't transpired that things are always much more complicated.
Perhaps one of the biggest focus is kind of in the field at the moment and certainly receiving a high degree of investment is things like regenerative medicine and also healthy ageing.
From my perspective, I think that those are still very much in the research phase in terms of understanding how pathways develop, how they work towards each other, and probably not best suited or not best addressed by pharmaceuticals just yet.
But certainly, if we could tackle some of those diseases that really affects people like dementia, then that would be something I would think would be very worthwhile. Because that's something that affects a lot of people and kind of steals their later years. So, I think that's something that would be a strong push for me.
Stuart: So, you're thinking about maybe as a prophylactic use to kind of stave off the early stages of dementia?
Sam: Yeah, and some of those early diagnostics are getting a lot better. So, by tailoring that prophylactic treatment with much more early detection or genetic profiling that again, could really make a big difference.
And like I said earlier, if we are able to develop a drug that doesn't have horrible side effects that can be given prophylactically and chronically, then that will be a really kind of good thing.
Stuart: So, actually feels like the barrier is always going to be there, but actually that might be a good thing because it allows you to do targeting or it just means that you can choose the most appropriate intervention for the time. I don’t know, is that a good characterization?
Sam: Well, we definitely need the barrier, it's very good. It does its job, but the more we can understand about it, like you say, and choose appropriate interventions, then yeah, the better.
There is a whole wealth of diseases and conditions that are just not served for at the moment. So, if they could have their own treatments that are effective and acceptable to patients, then that would be great.
Stuart: I mean, there's some suggestions maybe from other guests on this podcast that you could create transient entry in the blood-brain barrier. So, if you are kind of coupling physical rupture in the barrier with PolyNaut delivery, does that opt for anything else, do you think?
Sam: Well, because we use the blood-brain barrier and we use its biological function to travel across, I don't know how effective it will be if we disrupt it. What we have seen, certainly people have seen in things like glioblastoma and neuro-oncology is that brain disease can then lead to disruption of the blood-brain barrier in itself, which then does allow other drugs to penetrate.
One of the challenges there is that there's not only the transport across the blood-brain barrier, it's the depth of travel and diffusion within the parenchyma to reach those target cells.
But like I was saying earlier, I think that there's probably not one technology to fix everything, although I probably shouldn't say that. I should probably say that PolyNaut will fix everything.
But I think it is important that people are working across all different bases to find things. And some of them will also work together. They'll potentiate one another.
Stuart: It's all about kind of different fits, right tool for the right job.
Sam: Yeah, exactly.
Stuart: Okay. Coming back down to reality and I suppose, what's feasible for SomaServe to achieve in the near term, what excites you most about the next couple of years? What gets you out of the bed in the morning?
Sam: Well, despite a number of people saying that, I've gone over to the dark side in business development and sort of corporate development, there's still data actually. It's sitting down with a team, one week you sit down, and they say, “Oh, this isn't working. We're going to have to try something else.”
The next week you sit down, they say, “Yeah, it's all working now. We've got this great data, look at this, how amazing.” And it's a proper sense of relation. It really gets everyone going.
And when you're in this small team, the small company, you really feel it all together. You're part of one team and it really makes a difference. So, it's great fun. You get the highs and the lows and you kind of have to work out how to navigate them.
[Music Playing]
Stuart: The blood-brain barrier has been for a long time, a very real barrier to progress in treating neurological disorders. But could we be seeing some cracks in the wall that would allow development of more effective treatments and improve our understanding of this most vital of organs?
Sam and his team are attempting to find passage utilising transcytosis, a biological process that provides transport across the blood-brain barrier. We might hope to see exploitation of other biological processes to reach the treatment site and SomaServe to continue pursuing development of their new formulations.
On the other hand, James is exploring the use of ultrasound to open up the blood-brain barrier for treatment. This is a great example of one of the innovative approaches’ scientists are deploying to create cheap, convenient treatment for those who need it.
We look forward to seeing how the field develops in the future. That's all for today. Thanks so much for joining us, and many thanks to both Sam and James for bringing their great experience to this episode.
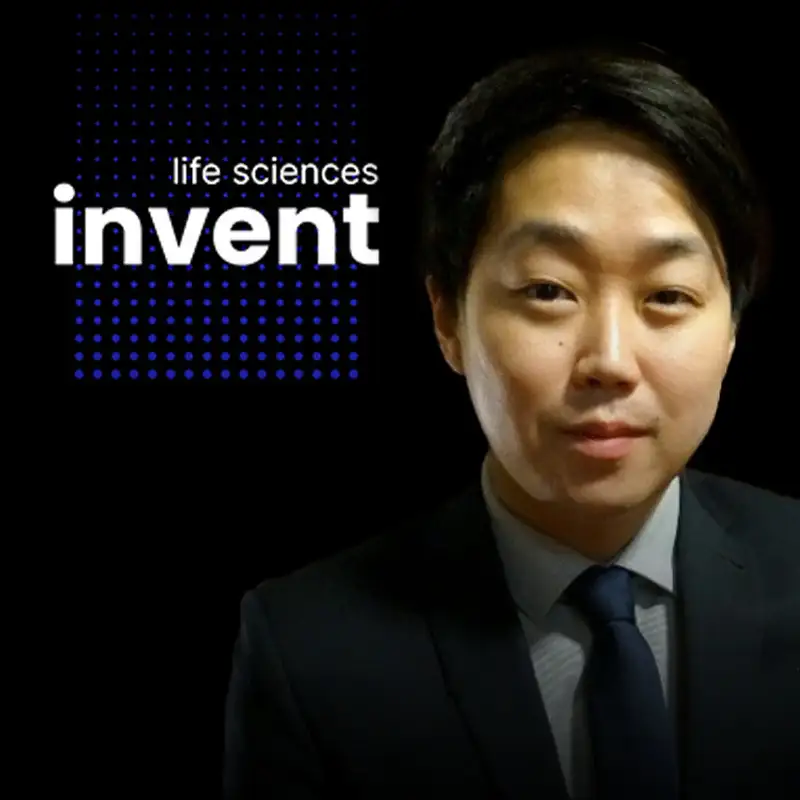